Communication is key. This is also true for everything that goes on in our body. Millions upon millions of proteins communicate through their so-called “active sites” either inside of our cells or outside of them, for example in our blood stream. The active site, which in the context of protein receptors is referred to as “binding site”, is probably the most important part of any protein, kind of its headquarters where business is done. Molecules are coming and going, docking into the site, being changed to deliver a message to the next protein, or simply being eliminated from the stream of communication. Once the proper communication flow inside of us gets interrupted, we feel it. For example, miscommunicating proteins in our joints cause us pain while working or even worse while resting. The root cause could be that one protein is not functioning properly and is sending the same message over and over again to the other proteins downstream of it. Interrupting this message flow could then be the key for restoring the original balance within our joints.
The active site of a protein that is involved in maintaining the right balance of joint tissue but leading to its destruction when its communication with other proteins gets out of balance, can be represented through a rendered image showing a red-grey opening that leads down to a grey tunnel. The two colors were picked randomly, although red usually represents a higher presence of polarity. In this context polarity is understood as a greater amount of electron-rich elements such as, for example, oxygen. The five red spheres in the entrance to the tunnel are oxygen atoms of water molecules that are found as stabilizing parts in all proteins. What looks like walls and a tunnel is in reality a complex backbone formed by 20 natural amino acids, which are the foundation of every protein. In the grey wall one can see this backbone as a long line with a curve or a ring structure and other sticks. All atoms in this backbone have the ability to interact with each other, thereby forming the 3D-structure and, more specifically, the active site.
How is it possible to visualize a protein and its active site like this? The technique leading to this spatial representation is the same that is used in all hospitals around the world to look for broken bones: X‑ray. All it takes is a crystal of the protein of interest and, by shooting X-ray beams through this crystal, one can visualize the positions of the heavier atoms and subsequently the whole structure of the protein. The element Hydrogen is too small to break X-ray beams, therefore the exact position of hydrogen atoms cannot be determined. However, knowing the rules of how many hydrogen atoms can be connected to any given element, it is obvious that for each of the red oxygen spheres, there must be always two hydrogen atoms around it: H2O.
Visualized X-ray structures of proteins are used by Medicinal Chemists to design molecules that fill the active site and subsequently restore the standard communication in our body. Thus, medicinal chemists work like molecular architects, who respond to the space of the active site by sculpting, fitting, and anchoring molecules, which subsequently can be developed into future medicines. They explore the active site like a room where some walls are completely rigid while others are flexible, allowing them to remodel this space. Since an X-ray structure provides information on the size and properties of the medicinal chemist’s construction site, it speeds up the design process immensely. However, for many proteins an X-ray structure is not available because they do not form suitable crystals. Without knowing the exact dimensions and characteristics of their active sites, the medicinal chemist conducts a more intuitive exploration with molecules that are probing the boundaries of the targeted space. This detective work naturally takes much longer since positive as well as negative binding data of up to hundreds of specially designed molecules will slowly establish a knowledge of the nature of the space.
As we can see in the rendered image, the grey tunnel is filled with a green molecule structure, green representing carbon (C) atoms. The mainly green structure also contains a few blue parts, blue for nitrogen (N) atoms, as well as red sticks symbolizing oxygen (O) atoms. The whole molecule is surrounded by a grey net that represents its electron density, which adds to its size and overall form. During the design phase, also known as Structure Activity Relationship (=SAR) Studies, which provide a constant data feedback loop on how the created molecules are interrupting the communication of the protein, the team realized that filling only the tunnel was not sufficient enough to prevent the protein from communicating (envision the green structure without the blue-green ring at the opening of the tunnel). The team concluded that the dimensions of the tunnel’s entrance would provide enough space to fit in an additional ring element. Ring structures, which can contain five or six atoms and are called five- or six-membered rings, are regularly used as building blocks. Knowing that a six-membered ring would bump into the red wall, led to the attachment of the smaller five-membered ring instead. Further knowing that the polar O-atoms inside the red wall would prefer N-atoms over neutral C-atoms, prompted the incorporation of blue N-atoms into the design. As a result, the new ring efficiently blocked the entrance space between the red wall and the tunnel. It also anchored the entire molecule in this very position by establishing a key interaction between the one N-atom and the red wall.
While the surfaces of the rendered image obstruct the view of the exact O-atom that is interacting with the N-atom of the five-membered ring, the wireframe image makes clear that it is the O-atom of an amino acid side chain. In fact, the anchor is formed between the N-atom of the ring and the manually inserted black H-atom – remember, H-atoms are too small to be visualized by X-ray beams - that is attached to the O-atom. This H-atom being bound to the more electron-rich O-atom is polarized through this bond, which subsequently enables it to partner with a high electron-density atom like the blue N-atom of the five-membered ring. This partnership exists solely through electronic attraction and not by forming an actual bond. The technical term for this specific interaction between the weaker H-atom and the stronger N-atom is “Hydrogen Bridge.” Medicinal chemists utilize the spectrum of forces in the atomic world to build these bridges in search for the perfect molecule. And while these bridges facilitate a new communication between the artificial molecule and the protein of interest, their greater purpose is to eventually help restore the healthy communication in our body.
Since the proteins in our bodies are not static crystals but dissolved and to a certain degree flexible structures, how do we know that one molecule is better suited as a medicine than others? To answer this crucial question about the value of a designed molecule, it needs to be further evaluated in an environment in which the protein is dissolved or ultimately in a living organism. Going “in vivo” and obtaining a positive answer from an animal with the same “human” disease that researchers are trying to cure, is the ultimate proof of a well-designed molecule. Such a molecule would not only bind perfectly to the disease-causing protein and subsequently trigger the desired biological function, it would also possess features to survive the body’s mechanisms to spot, identify, and eliminate foreign molecules. These crucial defense mechanisms are also governed and executed by proteins.
In summary, the communication system inside of us is extremely complex. The multitude of interdependent processes is unimaginable and ultimately controlled by an enormous apparatus of countless proteins working tirelessly together. As long as each protein keeps communicating in the context of its predefined set of communication pathways, we feel healthy. Just like in our external communication with family, friends, and others, a change in the code of conduct, for whatever reason, might cause misunderstandings, and in the worst case damage a connection irretrievably. Communication is key.
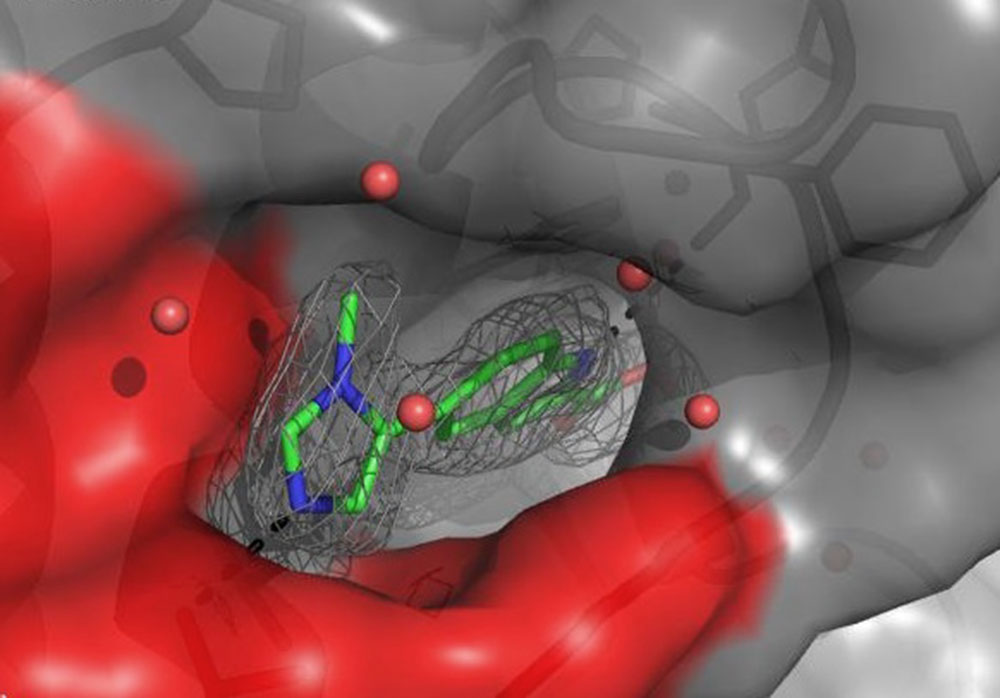
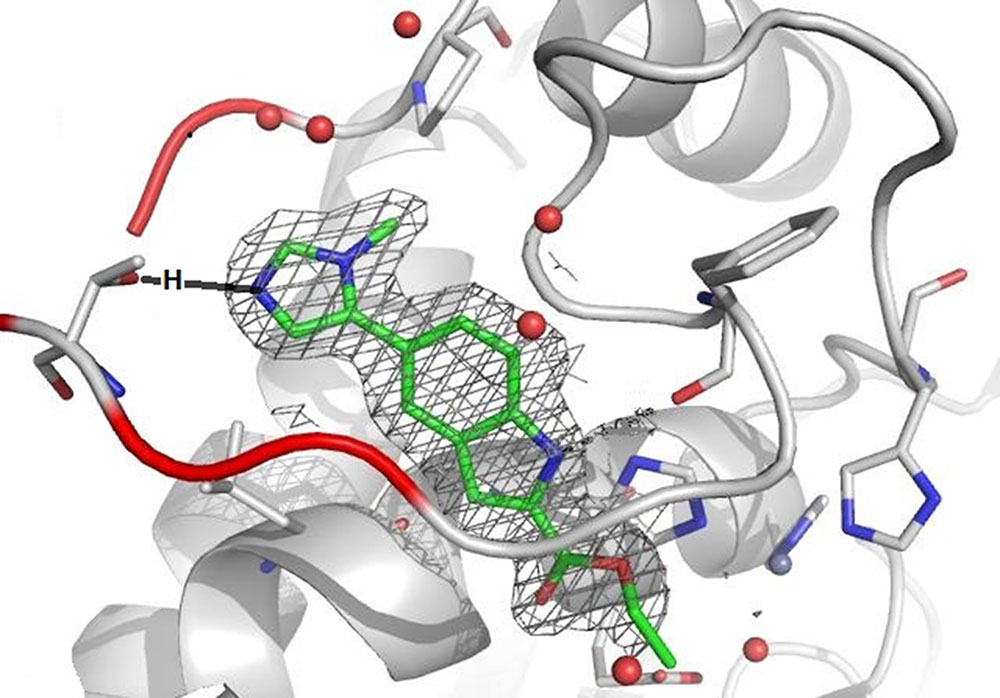
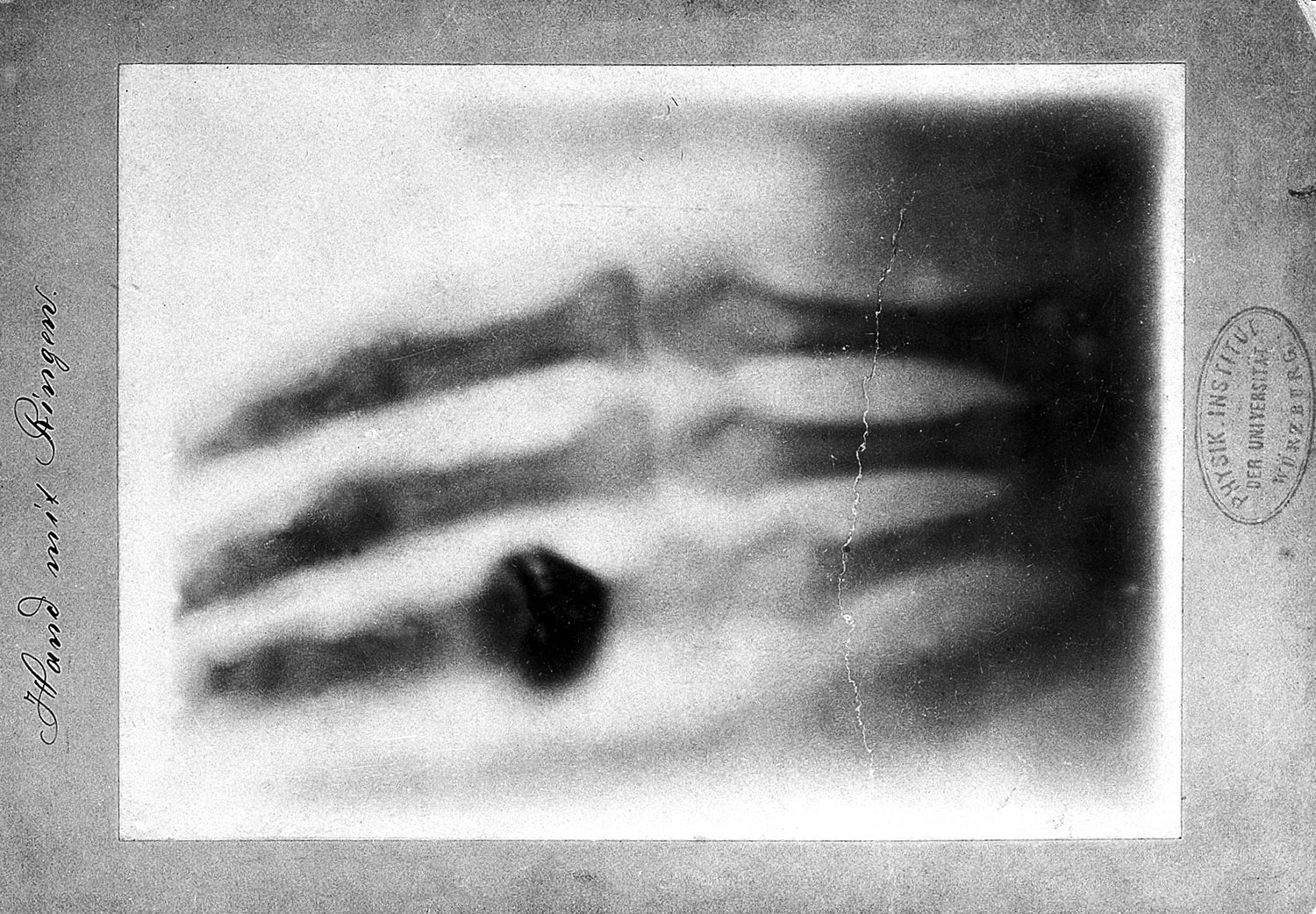
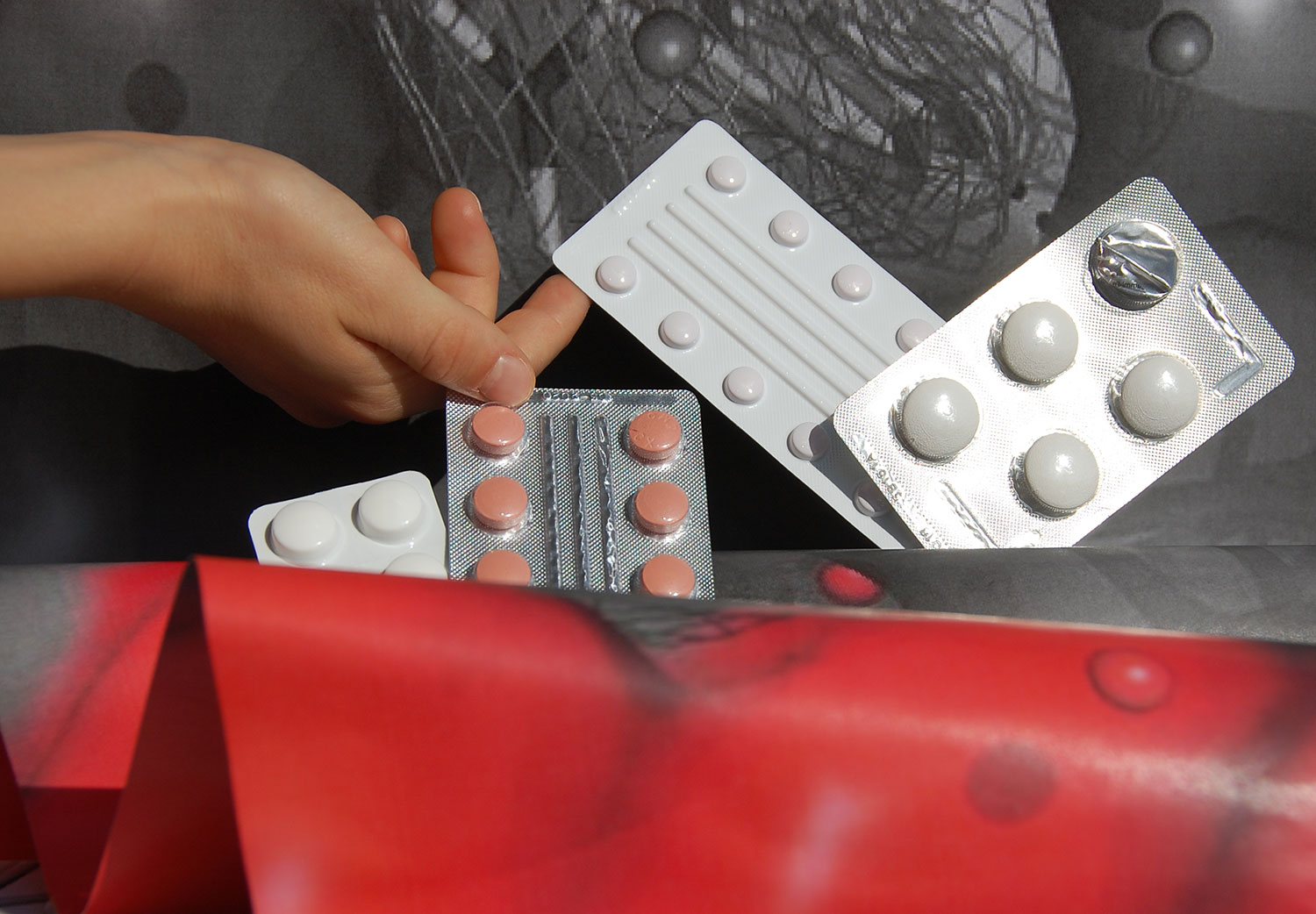